Welcome back to the world of medical engineering! Today, we delve into the fascinating realm of magnetic resonance imaging (MRI) and explore its properties and applications. In this two-part series, we will first explore the physics behind magnetic resonance and how it can be utilized to measure physical properties of tissues within the body. In the second part, we will delve into the intricacies of magnetic resonance imaging and learn how to translate the signals into image data. So, let’s unravel the mysteries behind this remarkable medical imaging technology.
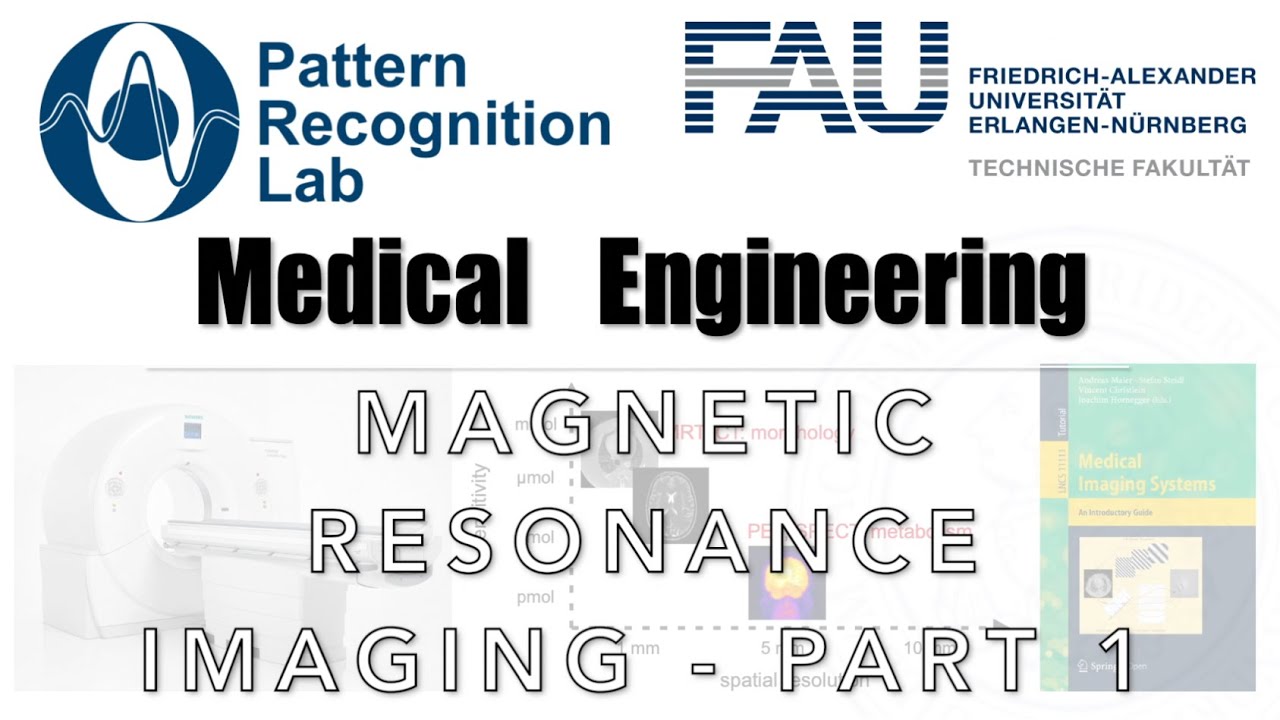
Contents
Understanding Magnetic Resonance
At the heart of MRI lies the principles of magnetic physics. The phenomenon relies on the interaction between varying magnetic fields and electromagnetic radiation. By comprehending these effects, we can unlock the potential of magnetic resonance imaging and harness it to understand and differentiate various tissues and their unique properties.
To harness the power of magnetic resonance, we require a strong external magnetic field, as the Earth’s magnetic field is not sufficient for our purposes. MRI scanners, therefore, house superconducting magnets that produce magnetic fields of 1.5 to 9 Tesla or more. These magnets are kept at extremely cold temperatures—close to absolute zero—to achieve superconductivity. The magnets are surrounded by gradient coils, which allow adjustments to the magnetic field, and the entire system is heavily shielded to prevent any interference.
It’s crucial to note that MRI scanners should be handled with extreme care due to their strong magnetic fields. Proper safety precautions must be taken to avoid accidents and potential harm to individuals or damage to the equipment.
The Magnetic Behavior of the Human Body
Contrary to popular belief, the human body does contain elements that exhibit magnetic behavior. In particular, hydrogen atoms within the body can be likened to small magnets. However, their magnetic fields align randomly due to the weak Earth’s magnetic field. To align the hydrogen atoms in a specific direction, a strong external magnetic field is applied, allowing them to become aligned. This alignment forms the basis of the magnetization required for MRI imaging.
Magnetization and Relaxation
After aligning the hydrogen atoms using the external magnetic field, we can induce magnetization by applying a radio frequency (RF) pulse at the appropriate frequency. This RF pulse causes the hydrogen atoms to undergo precession, a circular motion around the main magnetic field. Once the RF pulse is switched off, the precession continues, gradually decreasing in amplitude until it returns to the equilibrium state aligned with the main magnetic field. This process is known as relaxation.
The relaxation process occurs differently in two main directions: the direction parallel to the main magnetic field (longitudinal direction) and the direction perpendicular to it (transverse direction). The relaxation in the longitudinal direction, or T1 relaxation, is slower and follows a curve that takes approximately 2.5 seconds to double and around 12.5 seconds to complete. On the other hand, relaxation in the transverse direction, or T2 relaxation, occurs much more rapidly, taking approximately 45 milliseconds to halve and around 225 milliseconds to complete. These different rates of relaxation form the basis of the contrast seen in MRI images.
Imaging Contrasts in MRI
By manipulating the timing of the RF pulse and the repetition time between pulses, we can create different MRI image contrasts. A short echo time (TE) and short repetition time (TR) result in a T1-weighted image, highlighting differences in T1 relaxation times. Conversely, a long echo time and long repetition time produce a T2-weighted image, emphasizing differences in T2 relaxation times.
Additionally, we can create a proton density-weighted image by using a short TE and a long TR, allowing us to visualize the number of hydrogen nuclei per voxel. These various image contrasts enable us to study different tissue properties and enhance diagnostic capabilities.
Conclusion
Magnetic resonance imaging has revolutionized modern medical diagnostics, allowing us to delve deep into the human body and gain valuable insights into its internal structure and function. Through careful manipulation of magnetic fields, RF pulses, and timing parameters, we can harness the power of magnetic resonance to create detailed images that aid in diagnostics and treatment planning.
In the second part of this series, we will delve into the intricacies of MRI image reconstruction and explore how we can translate the signals obtained into informative and detailed images. Stay tuned as we continue our journey into the world of medical engineering and magnetic resonance imaging.
FAQs
Q: What is the main principle of magnetic resonance imaging?
A: Magnetic resonance imaging relies on the interaction between magnetic fields and electromagnetic radiation to generate detailed images of the human body. By aligning hydrogen atoms in the body using a strong external magnetic field and inducing magnetization through radio frequency pulses, we can obtain information about tissues and their properties.
Q: How does relaxation play a role in MRI?
A: Relaxation refers to the process by which magnetization returns to equilibrium after an RF pulse is applied. T1 relaxation refers to relaxation in the direction parallel to the main magnetic field, while T2 relaxation occurs in the direction perpendicular to it. The differences in relaxation rates allow us to create image contrasts and visualize different tissue properties.
Q: What are the main types of image contrasts in MRI?
A: The main types of image contrasts in MRI are T1-weighted, T2-weighted, and proton density-weighted images. T1-weighted images highlight differences in T1 relaxation times, while T2-weighted images emphasize differences in T2 relaxation times. Proton density-weighted images provide information about the number of hydrogen nuclei per voxel.
Q: How are MRI images reconstructed?
A: The process of reconstructing MRI images involves using mathematical algorithms to analyze the signals obtained from the scanner and convert them into detailed images. This process takes into account various factors such as spatial resolution, signal strength, and image contrast.
For more information about MRI technology and medical engineering, visit Techal.
Contributed by Techal